Often when people think of the word "nuclear", they imagine bombs and explosions. But this is only one facet of the word which encompasses an extraordinary subject in chemistry. While being extremely fascinating and useful, nuclear chemistry also holds many dangerous applications. In this article, we will explore the fundamentals of nuclear chemistry, including radioactivity, types of radiation, half-life, transmutations, and much more.
Nuclear chemistry took its beginnings in 1896 when French chemist Antoine Henri Becquerel was studying uranium salts and their connection to radiation. With the discovery of x-rays only earlier as well as the fact that Becquerel had been studying the phosphorescence of uranium salts, Becquerel thought that, if exposed to sunlight, the uranium salts would absorb the visible light and re-emit it as x-rays. Since x-rays were able to penetrate black paper, he wrapped his photographic film in black paper and would see if the uranium could darken the film underneath. He also placed different objects on the covered film such as coins and metal cutouts in order to see the outline. The sunlight exposed uranium did in fact darken the film and you could make out the unclear outline of the objects. Becquerel took these results and concluded that his ideas were correct. He decided to gather more data and tried executing his experiment again. However, the weather was not cooperating and, without sunlight, Becquerel could not continue his experiment. So he left the uranium salts and the photographic film in a dark drawer overnight. The next day, he took them out and developed the film, expected to see only a little bit of darkening. What he did see shocked him. The uranium salts had left a clear outline of the metal object that was left on top of the film. At first, he thought it was just left-over effects from long-lasting phosphorescence. But he soon found that the same happened with non-phosphorescent uranium as well. He also believed that the rays emitted by the uranium were similar to x-rays. However, unlike x-rays, these rays could be deflected by electric and magnetic fields.

Outline of Maltese cross made by radiation from radioactive uranium.
Becquerel's associates Marie Curie and, her husband, Pierre Curie, also began studying the uranium. They showed that the uranium atoms were giving off rays which darkened the photographic film when absorbed. Marie Curie coined the term radioactivity to describe the process by which materials give off rays through nuclear reactions. The rays and particles given off by radioactive material are called radiation. But why is the energy released by nuclear reactions much greater than the energy released by chemical reactions? For starters, nuclear reactions differ from chemical reactions in many ways. During a chemical reaction, atoms lose and gain electrons in order to reach stability. However, in nuclear reactions, radioisotopes, the nuclei of unstable isotopes, undergo several changes in order to gain stability. These reactions emit a lot of energy. Another difference is how chemical reactions are affected by catalysts, pressure, or temperature while nuclear reactions are unaffected by changes in these as well as the compound in which the reaction is taking place. For these reasons, nuclear reactions cannot be sped up, slowed, or stopped.
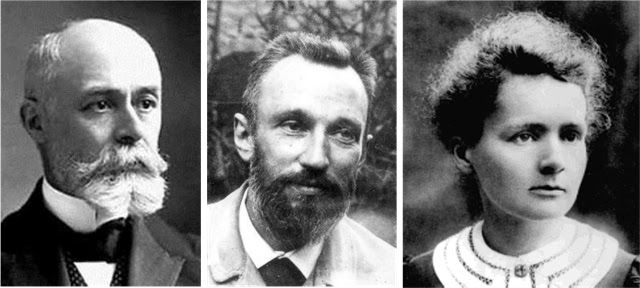
Antoine Henri Becquerel, Pierre and Marie Curie
In a radioactive atom, the size of the nucleus and the ratio of neutrons to protons determines the stability of the nucleus. Just like atoms in chemical reactions lose and gain electrons to become more stable, radioisotopes with less than ideal nuclei will lose some of their protons and neutrons. The unstable nucleus releases energy through radiation by the process of radioactive decay, in which the unstable radioisotopes are turned into stable isotopes of a different element. Essentially the nucleus spontaneously disintegrates into a different nucleus in order to reach stability. Since changes made to the protons of an atom affect its properties, the transition between an unstable radioisotope and a stable one also entails a transition between elements.
There are three main types of nuclear radiation emitted by radioactive decay: alpha radiation, beta radiation, and gamma radiation. Alpha radiation gets its name from the particles that it emits. Alpha particles are helium nuclei that have a mass number of 4 and an atomic number of 2. Since alpha particles have a charge of 2+, alpha radiation is positively charged. When a radioisotope emits alpha radiation, it is undergoing alpha decay. The alpha particles, with a large mass and charge, don't travel very far and have the least penetrating power of the three main types of radiation. Alpha radiation can be easily stopped by paper, clothing, or even the surface of your skin. On the other hand, while alpha radiation is positively charged, beta radiation is the opposite. During beta radiation, a neutron is split into a hydrogen proton and an electron. These electrons are called beta particles. Beta radiation has a slightly greater penetrating power than alpha radiation but can be stopped by metal foil or wood. Then there's gamma radiation. Gamma radiation is neither negatively nor positively charged. In fact, it has no charge at all. This is because, during gamma radiation, a high-energy photon, called a gamma-ray, is emitted. They are electromagnetic radiation and are often accompanied by alpha or beta particles as well. Since gamma rays themselves are just energy, they do not affect the atomic number or mass number of the radioisotope from which they are being emitted. Also, since gamma radiation has no mass or charge, it is extremely penetrating but can be stopped by lead or several meters of concrete.
But what is the role of radiation in nuclear reactions? Well, the protons in all nuclei repel each other and the only thing that holds them and the neutrons together is called the nuclear force, which is an attractive force that, in spite of magnetic repulsion, can hold together nuclear particles that are very close together. But, since the stability of nuclei depends on their size and the number of particles in them, nuclei that are too big will not be held together and are, therefore, unstable. Nuclei that are stable lie in the band of stability. Nuclei in the band of stability have ideal neutron-to-proton ratios. Based on this ratio, the unstable nuclei of radioisotopes will decay in certain ways. For example, nuclei with too many neutrons will emit beta particles through beta emission. Since beta particles are formed by the splitting of a neutron into a proton and an electron, beta emission helps to increase the number of protons while decreasing the number of neutrons.
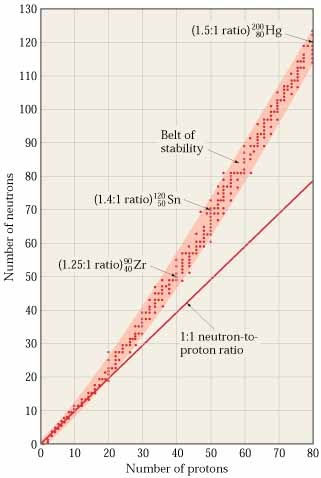
As the number of protons and neutrons increases, the ratio needed for stability increases. A 1:1 ratio does not hold for atoms with large nuclei.
Electron capture, which is the reverse of beta emission, occurs when there are too many protons. An electron is combined with a proton to create a neutron. This decreases the number of protons and increases the number of neutrons, stabilizing the neutron-to-proton ratio. Positron emission is another decay process that helps to increase the number of protons and decrease the number of neutrons. Positron emission involves a proton being changed into a neutron. It occurs when a positron, a positively charged particle with the mass of an electron, is emitted. When the atomic number of a nucleus is above 83, the nucleus is radioactive. With too many neutrons and protons, these nuclei undergo alpha emission in which alpha particles are emitted. The alpha radiation stabilizes the nuclei because it decreases the mass number by 4 and the atomic number by 2. But how do we measure the rate of a radioactive nuclei's decay? Half-life is the time it takes for half of a sample of a radioisotope to undergo decay. To put it into simpler terms, imagine you had 200 popcorn kernels. If it takes 30 seconds for 100 kernels to pop, then the half-life is 30 seconds. After 30 seconds 100 of the kernels will not have been popped. After another 30 seconds, half of the leftover kernels will be popped. So after 60 seconds, 50 of the original 200 kernels will not have been popped. After another 30 seconds, 25 kernels will not have been popped. Although it seems like it should take less time when the amount of kernels is less, the time it takes for each kernel to pop is constant so, even though there are fewer kernels, all the kernels that will pop will take the same amount of time to do so. If we were to graph the rate of decay we would see the half-life is exponential decay.
Scientists can use the predictability of radioactive decay for estimating the age of material that contain radioactive substances. They do this by finding the ratio of stable isotope to unstable isotope in the object and by using the half-life of the unstable isotope to see how much of it has decayed. When scientists are trying to find the age of the remains of an organism, they find the amount of carbon-4 it contains. Since the Earth's supply of carbon is constantly being replenished, the ratio of unstable carbon-14 to other stable carbon isotopes remain pretty constant. The predictability of the decay allows scientists to estimate the organism's age.
As earlier stated, when the number of protons in an atom is changed, the element changes as well. But what is this conversion called? A transmutation is the conversion of an atom of one element to an atom of another element. Two ways that transmutation can occur are by radioactive decay or when the nucleus of an atom is bombarded by protons, neutrons, or alpha particles. Transmutation can occur in nature or in a lab. In 1919, when Ernest Rutherford bombarded nitrogen atoms with alpha particles, he performed the first artificial transmutation. The bombardment produced a radioisotope that quickly decayed into stable oxygen and a proton.
But say the nuclei of some isotopes were to be bombarded by neutrons. In certain cases, these isotopes undergo fission in which the nucleus is split into fragments. When these fissionable atoms are struck by a neutron and undergo fission, they release two new atoms that may be of different elements as well as several neutrons and some energy. These neutrons continue the fission by striking other fissionable atoms until they all have undergone fission. For this reason, nuclear fission is a chain reaction that can release extremely large amounts of energy. But, in order to utilize this energy, the reaction must be controlled so that the energy will be released slowly. A nuclear reactor can be used to control the reactions as well as produce useful energy. It does this through neutron moderation and neutron absorption. In a nuclear reactor, there is a reaction chamber in which these processes take place. In this reaction chamber, there are fuel rods that are made of the fissionable isotope, control rods made of materials that absorb neutrons, and moderator rods containing graphite or water that slow neutrons. In neutron moderation, the neutrons are slowed so that they can be absorbed by the fuel rods for the reaction to continue. Without the moderation rods, the neutrons would travel to fast to be absorbed by the nuclei. Since the rate of fission will depend on how many neutrons strike the fuel rods, neutron absorption is necessary to decrease the number of neutrons in the reaction chamber. If the reaction were to go too fast, the reactor would overheat causing a meltdown. Consequently, control rods that absorb neutrons are essential when controlling the rate of fission. But how can we use all this energy released through fission? Well, the energy from the fission is released in the form of heat. This heat is used to produce steam that will drive a turbine. This turbine drives a generator in order to produce electricity that we can use to power things like our devices and our homes.
On the other hand, the opposite of fission is fusion. Unlike fission, fusion involves smaller nuclei combining into greater one of greater mass. A prime example of nuclear fusion can be found just by looking up. The sun, Earth's biggest source of energy, is a hot spot of fusion. All of the energy that we get from the sun is a result of nuclear fusion that is constantly taking place. On the sun, hydrogen nuclei fuse together producing a helium nucleus as well as positrons. This reaction releases far more energy than nuclear fission and explains how the sun has so much energy. So why don't we use fusion to power the world? Although this seems like an appealing alternative to fossil fuels and other sources of energy that we use, fusion only occurs at extremely high temperatures. It is very difficult to reach these temperatures in a controlled reaction. But, if we were to figure out a way to perform a controlled fusion, we would be able to switch from harmful fossil fuels that destroy the environment. The applications would be endless.
TL;DR (Too Long; Didn't Read)
Radioactivity - the process by which materials give off rays through nuclear reactions
Radiation - the rays and particles given off by radioactive material
Radioisotopes - the nuclei of unstable isotopes
Radioactive decay - the process by which the unstable radioisotopes are turned into stable isotopes of a different element
Alpha particles - helium nuclei that have a mass number of 4 and an atomic number of 2. They are given off during alpha radiation.
Beta particles - electron that is formed when a neutron is split into a hydrogen proton and an electron
Gamma-ray - a high-energy photon emitted during gamma radiation
Nuclear force - an attractive force that, in spite of magnetic repulsion, can hold together nuclear particles that are very close together
Band of stability - elements with a stable ratio of the number of neutrons to the number of protons in the nucleus lie in the band of stability
Electron capture - when there are too many protons, an electron is combined with a proton to create a neutron, decreasing the number of protons, increasing the number of neutrons, and stabilizing the neutron-to-proton ratio.
Positron emission - decay process which involves a proton being changed into a neutron. It helps to increase the number of protons and decrease the number of neutrons.
Positron - a positively charged particle with the mass of an electron emitted during positron emission decay
Half-life - the time it takes for half of a sample of a radioisotope to undergo decay
Transmutation - the conversion of an atom of one element to an atom of another element
Fission - fissionable atoms are struck by a neutron releasing two new atoms that may be of different elements as well as several neutrons and some energy (the nucleus is split into fragments)
Neutron moderation - neutrons are slowed so that they can be absorbed by the fuel rods for the reaction to continue
Neutron absorption - decreases the number of neutrons in the reaction chamber when control rods absorb the neutrons
Fusion - a process which involves smaller nuclei combining into greater one of greater mass and large amounts of energy being released
References:
Wilbraham, Antony C. Prentice Hall Chemistry. Prentice Hall, 2008.
CrashCourse, director. Nuclear Chemistry: Crash Course Chemistry #38. YouTube, YouTube, 11 Nov. 2013, www.youtube.com/watch?v=KWAsz59F8gA.
“Fusion Basics.” Princeton Plasma Physics Lab, www.pppl.gov/about/fusion-basics.
Band of Stability Image Source: “21.2 Patterns of Nuclear Stability.” Chemistry: The Central Science, Chapter 21, Section 2, wps.prenhall.com/wps/media/objects/3313/3392670/blb2102.html.
Antoine Henri Becquerel, Pierre and Marie Curie Image Source: Serious Science
Becquerel's Experiment Image Source: “Benchmarks: Henri Becquerel Discovers Radioactivity on February 26, 1896.” EARTH Magazine, 27 Mar. 2014, www.earthmagazine.org/article/benchmarks-henri-becquerel-discovers-radioactivity-february-26-1896.
Written by Mahathi Somula
Comments